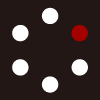
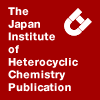
HETEROCYCLES
An International Journal for Reviews and Communications in Heterocyclic ChemistryWeb Edition ISSN: 1881-0942
Published online by The Japan Institute of Heterocyclic Chemistry
e-Journal
Full Text HTML
Received, 22nd August, 2009, Accepted, 22nd October, 2009, Published online, 22nd October, 2009.
DOI: 10.3987/COM-09-S(S)116
■ Catalytic Asymmetric 1,3-Dipolar Cycloaddition of Azomethine Imines to Allyl Alcohol Utilizing Tartaric Acid Ester as a Chiral Auxiliary
Katsuyoshi Tanaka, Tomomitsu Kato, Yutaka Ukaji,* and Katsuhiko Inomata*
Chemistry Course, College of Science and Engineering, School of Chemistry, Kanazawa University, Kakuma, Kanazawa, Ishikawa 920-1192, Japan
Abstract
The catalytic regio- and enantioselective 1,3-dipolar cycloaddition of azomethine imines to allyl alcohol was achieved by utilizing diisopropyl (R,R)-tartrate as a chiral auxiliary to afford the corresponding optically active trans-pyrazolidines with enantioselectivities up to 93% ee. Addition of MgBr2 was crucial to realize reproducible high enantioselectivity.The 1,3-dipolar cycloaddition is one of the most important methods for the construction of heterocyclic 5-membered rings in organic chemistry.1 The current challenge for 1,3-dipolar cycloaddition is to control the absolute configuration because the multiple chiral centers can be formed in a single step.2 Although a lot of cycloadditions of nitrone possessing nitrogen and oxygen atoms have been developed, the cycloaddition of azomethine imine which contains two nitrogen atoms was still limited.3 We have previously reported asymmetric 1,3-dipolar cycloadditions of nitrile oxides and nitrones utilizing tartaric acid ester as a chiral auxiliary.4,5 Recently we also developed an asymmetric 1,3-dipolar cycloaddition of azomethine imines to allyl alcohol utilizing a stoichiometric amount of diisopropyl (R,R)-tartrate [(R,R)-DIPT] as a chiral auxiliary.6 Toward improvement of this method, we made efforts to establish the catalytic reaction system. Herein, we describe the regio- and enantioselective 1,3-dipolar cycloaddition of azomethine imines using a catalytic amount of (R,R)-DIPT as a chiral auxiliary.
First, the 1,3-dipolar cycloaddition of an azomethine imine, 1-benzylidene-3-oxopyrazolidin-1-ium-2-ide (2a), was examined using 0.2 equivalent of (R,R)-DIPT in MeCN at 80 °C: To a mixed solution of allyl alcohol (1) and 0.2 equivalent of (R,R)-DIPT in MeCN were added 1.4 equivalents of MeMgBr and azomethine imine 2a successively at 0 °C.6 After heating at 80 °C for 2 d, the corresponding trans-pyrazolidine 3a was obtained with moderate enantioselectivity (Table 1, Entry 1). Production of regioisomer and/or diastereomer was not confirmed. When the azomethine imine 2a was slowly added into the reaction mixture, no enhancement of the enantioselectivity was observed (Entry 2). The solubility of azomethine imine 2a is low in less polar solvent. Thus, the azomethine imine 2a might gradually dissolve in the less polar organic solvent to be supplied into the reaction system with progress of the reaction, that is, 0.2 equivalent of bis(bromomagnesium)salt of (R,R)-DIPT could act just like a stoichiometric catalyst toward slightly dissolved 2a. Actually, when benzene was used as a cosolvent, the reaction mixture was heterogeneous, but enantioselectivity was slightly enhanced (Entry 3). The use of toluene or cC5H9OMe as a cosolvent did not improve the enantioselectivity (Entries 4 and 5). The pyrazolidine 3a was obtained in 75% ee in MeCN/ClCH2CH2Cl even the reaction mixture was homogeneous (Entry 6). Though other cosolvents were widely examined, improvement of the enantioselectivity was not observed any more (for example, Entry 7). These results indicated that slow loading of the azomethine imine into the reaction mixture was not so effective as we initially expected.
Next, the effect of Lewis acid was investigated, since it was expected to work as an activator of the asymmetric 1,3-dipolar cycloaddition under chiral reaction field derived from magnesium salt of (R,R)-DIPT or a promoter of the exchange of the magnesium salt of the produced pyrazolidine for the magnesium salt of allyl alcohol.7 The reaction was carried out by an alternative procedure: Azomethine imine 2a was treated with Lewis acid, followed by subsequent addition of allyl alcohol (1), (R,R)-DIPT, and Grignard reagent as shown in Table 2. The reproducible higher enantioselectivity was realized when the 1,3-dipolar cycloaddition was performed in the presence of an equimolar amount of MgBr28 in EtCN (Entry 2). The enantioselectivity was rather fluctuated in the absence of MgBr2 even by this addition procedure.9 Pre-treatment of azomethine imine 2a with MgBr2 made 2a soluble in the solvent by formation of MgBr2 complex to realize the reproducible results. When the amount of MgBr2 was reduced to half, enantioselectivity decreased (Entry 3). Furthermore, the use of alkylmagnesium chloride as a Grignard reagent to generate magnesium alkoxides improved the enantioselectivity remarkably (Entry 4). The concentration of the Grignard reagent scarcely influenced the enantioselectivity (Entries 4 and 5). Influence of anion in the magnesium salt was next investigated. MgCl2 was found to be also effective, though it did not dissolve completely in the reaction mixture (Entry
9). MgCl2 worked well even when MeMgBr was used to generate magnesium alkoxides (Entry 10). MgI2 and Mg(OTf)2 were not so effective compared with MgBr2 (Entries 11 and 12). Mg(ClO4)2 showed poor enantioselectivity (Entry 13). Furthermore, the enantioselectivity was slightly influenced by the concentration of the reactants (Entries 5–8). The cycloaddition under much concentrated conditions afforded 3a with lower enantioselectivity (Entry 8). Due to simplicity and reproducibility of the procedure, the following reactions were carried out by the use of MgBr2 as a Lewis acid.10
The catalytic asymmetric cycloaddition of several azomethine imines 2 to allyl alcohol (1) was performed in the presence of MgBr2 as shown in Table 3.11 Aryl-substituted azomethine imines 2b and 2c realized high enantioselectivities (Entries 2 and 3). The cycloaddition of pentyl- and cyclohexyl-substituted azomethine imines 2d and 2e also proceeded in an enantioselective manner (Entries 4 and 5). t-Butyl-substituted azomethine imine 2f resulted in the highest enantioselectivity of 93% ee (Entry 6).
The absolute configuration of 3a was previously determined to be R,R by chemical correlation.6 The absolute configurations of other products were tentatively determined to be R,R for 3b, 3c, 3e, and 3f and 5S,7R (configurational arrangement of the substituents at 5- and 7-positions is same as other products) for 3d, respectively.
Although the precise reaction mechanism is not yet clear, a plausible mechanism of the catalytic cycle is shown in Scheme 1. In this system, the dramatic rate enhancement was not observed by the addition of magnesium salt. The salt might promote the replacement of the produced magnesium-bridging chiral salt (E) by the magnesium salt of 1 (B)13 and azomethine imine complex with magnesium salt (C) to release a magnesium bridging salt (F) from the catalytic cycle smoothly. Furthermore, there might be another possibility that magnesium salt diminishes the direct uncatalyzed racemic reaction between B and C into F through the tight complexation of the azomethine imine with magnesium salt by reducing the dipolar nature of the azomethine imine. However, the present reaction was found not to be the case, because the reaction without the magnesium tartrate proceeded as well.14
As described above, an attractive catalytic asymmetric cycloaddition of azomethine imines to allyl alcohol has been developed. Because of easy availability of (R,R)- and (S,S)-DIPT, this method provides the useful way to prepare both enantiomers of trans-pyrazolidines.
ACKNOWLEDGEMENTS
The present work was financially supported in part by a Grant-in-Aid for Scientific Research on Priority Areas “Advanced Molecular Transformations of Carbon Resources” from The Ministry of Education, Culture, Sports, Science and Technology (MEXT) and a Grant-in-Aid for Scientific Research (C) from Japan Society for the Promotion of Science (JSPS).
References
1. A. Padwa, 'Comprehensive Organic Synthesis,' Vol. 4, ed. by B. M. Trost and I. Fleming, Pergamon Press, New York, 1991, pp. 1069-1109.
2. K. V. Gothelf, 'Cycloaddition Reactions in Organic Synthesis,' ed. by S. Kobayashi and K. A. Jørgensen, Wiley-VCH, Weinheim, 2002, Chapter 6; S. Kanemasa, 'Cycloaddition Reactions in Organic Synthesis,' ed. by S. Kobayashi and K. A. Jørgensen, Wiley-VCH, Weinheim, 2002, Chapter 7; L. M. Stanley and M. P. Sibi, Chem. Rev., 2008, 108, 2887. CrossRef
3. S. Kobayashi, H. Shimizu, Y. Yamashita, H. Ishitani, and J. Kobayashi, J. Am. Chem. Soc., 2002, 124, 13678; CrossRef R. Shintani and G. C. Fu, J. Am. Chem. Soc., 2003, 125, 10778; CrossRef Y. Yamashita and S. Kobayashi, J. Am. Chem. Soc., 2004, 126, 11279; CrossRef S. Shirakawa, P. J. Lombardi, and J. L. Leighton, J. Am. Chem. Soc., 2005, 127, 9974; CrossRef A. Suárez, C. W. Downey, and G. C. Fu, J. Am. Chem. Soc., 2005, 127, 11244; CrossRef W. Chen, X.-H. Yuan, R. Li, W. Du, Y. Wu, L.-S. Ding, and Y.-C. Chen, Adv. Synth. Catal., 2006, 348, 1818; CrossRef H. Suga, A. Funyu, and A. Kakehi, Org. Lett., 2007, 9, 97; CrossRef W. Chen, W. Du, Y.-Z. Duan, Y. Wu, S.-Y. Yang, and Y.-C. Chen, Angew. Chem. Int. Ed., 2007, 46, 7667; CrossRef M. P. Sibi, D. Rane, L. M. Stanley, and T. Soeta, Org. Lett., 2008, 10, 2971. CrossRef
4. Y. Ukaji, K. Sada, and K. Inomata, Chem. Lett., 1993, 22, 1847; CrossRef M. Shimizu, Y. Ukaji, and K. Inomata, Chem. Lett., 1996, 25, 455; CrossRef Y. Yoshida, Y. Ukaji, S. Fujinami, and K. Inomata, Chem. Lett., 1998, 27, 1023; CrossRef Y. Ukaji, M. Ima, T. Yamada, and K. Inomata, Heterocycles, 2000, 52, 563; CrossRef M. Tsuji, Y. Ukaji, and K. Inomata, Chem. Lett., 2002, 31, 1112; CrossRef Y. Ukaji and K. Inomata, Synlett, 2003, 1075. CrossRef
5. Y. Ukaji, K. Taniguchi, K. Sada, and K. Inomata, Chem. Lett., 1997, 26, 547; CrossRef D. Xia, K. Taniguchi, Y. Ukaji, and K. Inomata, Chem. Lett., 2001, 30, 468; CrossRef D. Xia, Y. Ukaji, S. Fujinami, and K. Inomata, Chem. Lett., 2002, 31, 302; CrossRef D. Xia, K. Taniguchi, Y. Hamamoto, K. Sada, S. Fujinami, Y. Ukaji, and K. Inomata, Bull. Chem. Soc. Jpn., 2006, 79, 1069. CrossRef
6. T. Kato, S. Fujinami, Y. Ukaji, and K. Inomata, Chem. Lett., 2008, 37, 342. CrossRef
7. It was reported that magnesium salts accelerated 1,3-dipolar cycloaddition of nitrile oxides and nitrones to allylic alcohols: S. Kanemasa, T. Tsuruoka, and E. Wada, Tetrahedron Lett., 1993, 34, 87; CrossRef S. Kanemasa, M. Nishiuchi, A. Kamimura, and K. Hori, J. Am. Chem. Soc., 1994, 116, 2324; CrossRef S. Kanemasa, T. Tsuruoka, and H. Yamamoto, Tetrahedron Lett., 1995, 36, 5019; CrossRef S. Kanemasa and T. Tsuruoka, Chem. Lett., 1995, 24, 49; CrossRef S. Kanemasa, K. Okuda, H. Yamamoto, and S. Kaga, Tetrahedron Lett., 1997, 38, 4095; CrossRef S. Kanemasa, Synlett, 2002, 1371. CrossRef
8. Magnesium bromide was generated in situ by the treatment of magnesium metal with 1,2-dibromoethane in THF.
9. The cycloaddition in the absence of MgBr2 in MeCN once gave 3a in 52% yield with 63% ee. However, in the worst case, 3a was produced in 66% yield with 35% ee.
10. Not only magnesium salts but also other metal salts were examined. However, the chemical yields and/or the enantioselectivity of the product 3a were not satisfactory as follows. Chemical yield/ee; Zn(OTf)2: 41%/15% ee, CuCl2: 9%/80% ee, Cu(OTf)2: 4%/57% ee, NiCl2: 52%/66% ee, Cp2TiCl2: 17%/93% ee, Cp2ZrCl2: 17%/85% ee.
11. By comparison of the results between from the reaction of 1.0 mmol scale of 2 in 6 ml EtCN and from the reaction of 0.5 mmol scale of 2 in 6 ml EtCN, the better results are listed in Table 3.
12. By-product 4 might be produced by 1,3-dipolar cycloaddition of 2d to an enamine-type tautomer of 2d generated in situ.
13. The 1,3-dipolar cycloaddition of 2a to ally benzyl ether did not proceed at all under similar reaction conditions. The generation of chloromagnesium salt of allyl alcohol (1) (B) was crucial for the present reaction.
14. A background reaction in the absence of chloromagnesium salt of (R,R)-DIPT gave racemic 3a in 65% yield under the similar conditions.