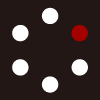
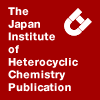
HETEROCYCLES
An International Journal for Reviews and Communications in Heterocyclic ChemistryWeb Edition ISSN: 1881-0942
Published online by The Japan Institute of Heterocyclic Chemistry
e-Journal
Full Text HTML
Received, 8th June, 2010, Accepted, 24th June, 2010, Published online, 25th June, 2010.
DOI: 10.3987/COM-10-11988
■ A New Synthetic Strategy for 2-Deoxy-D-ribose via Palladium(II)-Catalyzed Cyclization of Aldehyde
Masahiro Miyazawa,* Ken-ichiro Awasaguchi, Ikuyo Uoya, Hajime Yokoyama, and Yoshiro Hirai*
Department of Chemistry, Graduate School of Science and Engineering, Toyama University, Gofuku 3190, Toyama 930-8555, Japan
Abstract
We achieved a total synthesis of 2-deoxy-D-ribose through intramolecular Pd(II)-catalyzed cyclization of aldehyde via an unstable hemiacetal intermediate as a key step.INTRODUCTION
Carbohydrates are the most abundant organic compounds in nature, and 2-deoxy-D-ribose is a constituent of DNA. 1-Substituted 2-deoxy-D-riboses also possess diverse biological activities.1 These compounds have been constructed in a wide variety of ways.2 We previously developed a stereoselective construction of polysubstituted heterocyclic compounds using palladium(II)-catalyzed intramolecular cyclization.3 We have also reported cyclization via a hemiacetal intermediate using a palladium(II) catalyst and found a stereoselective method for the construction of trisubstituted tetrahydropyran.4 We report here a synthesis of 2-deoxy-D-ribose by using this methodology.
RESULTS AND DISCUSSION
Our retrosynthetic analysis of 2-deoxy-D-ribose (1) is shown in Scheme 2. 2-Deoxy-D-ribose would be formed from the 4-vinylfuranoside 2. The construction of 2 could be achieved by palladium(II)-catalyzed heterocyclization of the aldehyde 3 and benzyl alcohol. The aldehyde 3 can be easily prepared from L-(-)-malic acid (4).
The synthesis of the key intermediate 3 is shown in Scheme 3. The benzylideneacetal 5 was prepared according to the reported procedure in two steps from L-(-)-malic acid (4).5 Oxidation of 5 by Swern oxidation followed by Horner-Wadsworth-Emmons reaction (triethyl phosphonoacetate, NaH) afforded the (E)-α,β-unsaturated ester 6 in 78% yield. Reduction (DIBALH) of 6 followed by protection of the resulting alcohol with DHP furnished the THP-ether 7. DIBALH reduction of 7 and oxidation of the resulting primary alcohol with Dess-Martin periodinane gave the aldehyde 3.6
We examined the cyclization of the aldehyde 3 with a variety of alcohols (Table 1). Initially, treatment of the aldehyde 3 with 5 mol% of PdCl2(MeCN)2 catalyst and 5 equiv. of MeOH in THF at room temperature furnished the cyclized products 2 as a 4.6 : 3.0 : 1.0 mixture of 2a : 2b : 2c in 40% isolated yield (entry 1). When i-PrOH was used, the ratio of 4R-isomer : 4S-isomer (2a + 2b : 2c) was increased to 10.8 : 1 (entry 2). However, when t-BuOH was used, no reaction was observed (entry 3), and bezyl alcohol was found to give the best result (entry 5). The ratio of 1R-isomer : 1S-isomer (2a + 2c : 2b) was almost 1.8 : 1 in any reaction conditions. On the other hand, 4R-isomer formed in the predominance more than 4S-isomer (4R-isomer : 4S-isomer = 6~11 : 1).
The stereochemistry of these diastereomers 2a~c could not be determined at this point. In order to determine the relative stereochemistry, the cyclized products 2a~c were converted to the corresponding primary alcohols 8a~c (Scheme 4). Our initial attempts to achieve double bond oxidation of the cyclized products 2a~c by ozonolysis led to low yields of the desired aldehyde. After several attempts, we found that oxidation of 2a~c proceeded smoothly in the presence of sodium periodate and a catalytic amount of osmium tetroxide in dioxane / H2O to give the corresponding aldehydes. Treatment of these aldehydes with NaBH4 in MeOH gave the primary alcohols 8a~c in moderate yield for the two-step sequence. The alcohols 8a~c could be separated by silica gel column chromatography.
The relative stereochemistry of 8a~c was established by NOE experiments. For example, as shown in Figure 1, NOE correlations were observed between the C-3 hydrogen and the C-2 β-hydrogen, and between the C-2 β-hydrogen and the C-1 hydrogen.
The stereochemistry of the cyclized products formed in the palladium-catalyzed cyclization depends on various factors. A plausible mechanism for the cyclization of the aldehyde 3 is shown in Scheme 5. Initially, the aldehyde 3 is converted into the alcohol 9 (step 1). The hemiacetal intermediate is formed by 1,2-addition of benzyl alcohol to the aldehyde 9 (step 2). The olefin and hydroxyl groups of the allylic alcohol and hemiacetal coordinate with the palladium complex (step 3). The resulting complex may be present as an equilibrium mixture of four forms (TS1, TS2, TS3, and TS4). TS1 and TS2 are favored over TS3 and TS4 owing to the anomeric effect of the hemiacetal oxygen. On the other hand, TS2 and TS4 are strongly destabilized by A1,3 strain. Because of these two factors, the overall mechanism can account for the observed predominant formation of 2a and 2b.
Finally, deprotection of all benzyl groups in 8a and 8b was conducted at an atmospheric pressure of hydrogen in EtOH with palladium over charcoal (10%). After 58 h, 2-deoxy-D-ribose was obtained in good yield. The product was easily purified by silica gel column chromatography. The product was identical to an authentic sample of 2-deoxy-D-ribose in all respects, including the optical activity (Table 2).
In summary, we have developed an efficient synthesis of 2-deoxy-D-ribose via palladium(II)-catalyzed cyclization. The synthesis involved 12 steps and proceeded in good overall yield from L-(-)malic acid.
EXPERIMENTAL
General Remarks: All moisture sensitive reactions were carried out under an argon atmosphere. Anhydrous solvents were obtained as follows: tetrahydrofuran (THF) and dichloromethane were purchased from Kanto Chemical Co., Ltd.; N,N-dimethylformamide (DMF) and dimethyl sulfoxide (DMSO) was distilled from CaH2. Triethylamine was distilled from KOH. Column chromatography was performed with Silica gel 60N and Fuji BW-820. Analytical thin layer chromatography (TLC) was conducted on precoated TLC plates (silica gel 60F254, Merck) visualized under UV light and stained with either phosphomolybdic acid or p-anisaldehyde. Melting points were determined with Yanaco micro melting point apparatus and were uncorrected. IR spectra were measured with a JASCO Model FT/IR-6100 spectrometer. Mass spectra (MS) and high resolution mass spectra (HRMS) were recorded at a JEOL JMS-700 or JMS-T100TD spectrometer. Elemental analyses were performed on a Yanaco MT-5. Optical rotations ([α]D) were determined with a JASCO P-1020 polarimeter. 1H NMR spectra were recorded at 300 MHz with a JEOL JNM-ECX 300 spectrometer or 600 MHz with a JEOL JNM-ECP 600 using tetramethylsilane (TMS) as the internal standard (0.00 ppm). Chemical shifts were reported in ppm (δ) downfield from TMS. The following abbreviations were used to explain the multiplicities: s = singlet, d = doublet, t = triplet, m = multiplet. 13C NMR spectra were recorded at 75 MHz with a JEOL JNM-ECX 300 spectrometer with chemical shifts reported in ppm (δ).
(S)-Butane-1,2,4-triol
To a solution of borane dimethyl sulfide complex (42.4 mL, 0.45 mol) and trimethyl borate (49.9 mL, 0.45 mol) was added a solution of L-(-)-malic acid (4) (20.0 g, 0.15 mol) in THF (100 mL) at 0 oC under an argon atmosphere. The mixture was stirred at 0 oC for 40 min, then warmed to rt and stirred for 7 h. The reaction was quenched with MeOH and concentrated in vacuo. The residue was purified by silica gel column chromatography (CH2Cl2-EtOH = 10:1 to 1:1, gradient) to afford (S)-butane-1,2,4-triol (16.1 g, >99 %yield) as a colorless oil.
triol; colorless oil; [α]20D -29.4 (c 0.94, MeOH); 1H NMR (300 MHz, CD3OD) δ 3.79-3.63 (m, 3H), 3.52-3.40 (m, 2H), 1.78-1.50 (m, 2H); 13C NMR (75 MHz, CD3OD) δ 70.7, 67.5, 59.9, 37.1; IR (neat, cm-1) 3341, 2940, 2884, 1421, 1060; MS (EI) m/z 76(5), 75(100), 61(9), 60(6), 58(20), 57(66), 55(5);
(DART) m/z 107 ([M+H]+); HRMS-DART calcd. for C4H11O3 ([M+H]+) 107.0708, found 107.0717.
((2S,4S)-2-Phenyl-1,3-dioxan-4-yl)methanol (5)
To a suspension of the triol (201 mg, 1.89 mmol) in CH2Cl2 (19 mL) was added benzaldehyde dimethyl acetal (0.31 mL, 2.08 mmol) and p-toluenesulfonic acid (2 mg, 0.01 mmol) at rt under an argon atmosphere, then the mixture was vigorously stirred at the same temperature for 24 h. The reaction was quenched with Et3N and concentrated in vacuo. The residue was purified by silica gel column chromatography (hexane-AcOEt = 10:1 to 1:1, gradient) to afford ((2S,4S)-2-phenyl-1,3-dioxan-4-yl)methanol (5) (314 mg, 85% yield in 2 steps) as a white solid.
5; white solid; mp 58.0-60.0 oC; [α]20D 10.6 (c 0.59, CHCl3); 1H NMR (300 MHz, CDCl3) δ 7.54-7.46 (m, 2H), 7.42-7.34 (m, 3H), 5.55 (s, 1H), 4.31 (ddd, J = 11.1, 5.2, 1.2 Hz, 1H), 4.09-3.94 (m, 2H), 3.77-3.61 (m, 2H), 2.00-1.86 (m, 1H), 1.46 (dtd, J = 13.1, 2.5, 1.4 Hz, 1H); 13C NMR (75 MHz, CDCl3) δ 138.3, 129.0, 128.3(2C), 126.1(2C), 101.3, 77.5, 66.6, 65.7, 26.8; IR (KBr, cm-1) 3438, 2934, 2912, 1458, 1414, 1240, 1133, 1097, 1065, 1022, 969, 764, 704; MS (EI) m/z 194(M+, 16), 193(13), 163(14), 123(11), 122(12), 106(31), 105(84), 78(65), 77(100), 57(40), 51(27); (DART) m/z 195 ([M+H]+); HRMS-DART calcd. for C11H15O3 ([M+H]+) 195.1021, found 195.0997.
(2S,4S)-2-Phenyl-1,3-dioxane-4-carbaldehyde
To a solution of dimethyl sulfoxide (1.1 mL, 15.4 mmol) in CH2Cl2 (15 mL) was added oxalyl chloride (0.67 mL, 7.72 mmol) at -78 oC under an argon atmosphere. After stirring at the same temperature for 15 min, to the reaction mixture was added a solution of ((2S,4S)-2-phenyl-1,3-dioxan-4-yl)methanol (5) (1.00 g, 5.15 mmol) in CH2Cl2. The suspension was stirred at -78 oC for 20 min, then the mixture was added Et3N (3.2 mL, 23.2 mmol) at -78 oC and warmed to rt. After stirring for 20 min, the reaction mixture was poured into ice and 1 N HCl, and the aqueous phase was extracted with CHCl3 (x 2). The combined organic layers were washed with saturated aqueous NaHCO3 solution and brine, filtered, and concentrated in vacuo to afford (2S,4S)-2-phenyl-1,3-dioxane-4-carbaldehyde (1.16 g) which was used immediately without further purification. An aliquot was purified by silica gel column chromatography (hexane-AcOEt = 3:1) to afford an analytical pure sample as a colorless oil.
aldehyde; colorless oil; [α]20D -63.2 (c 0.90, CHCl3); 1H NMR (300 MHz, CDCl3) δ 9.74 (s,1H), 7.60-7.30 (m, 5H), 5.61 (s, 1H), 4.41-4.33 (m, 2H), 4.02 (td, J = 11.9, 2.8 Hz, 1H), 2.06-1.91(m, 1H), 1.81(dtd, J = 13.4, 3.0, 1.5 Hz, 1H); 13C NMR (75 MHz, CDCl3) δ 200.6, 137.6, 129.2, 128.4(2C), 126.1(2C), 101.2, 80.4, 66.5, 25.9; IR (neat, cm-1) 3455, 2970, 2858, 1737, 1397, 1375, 1138, 1105, 996, 759, 700; MS (EI) m/z 192 (M+, 2), 191 (7), 163(100), 107(40), 105(64), 91(42), 79(66), 77(56), 57(22), 51(17); (DART) m/z 193 ([M+H]+); HRMS-DART calcd. for C11H13O3 ([M+H]+) 193.0865, found 193.0864.
(E)-Ethyl 3-((2S,4S)-2-phenyl-1,3-dioxan-4-yl)acrylate (6)
To a suspension of sodium hydride (60% in oil, 288 mg, 7.21 mmol) in THF (20 mL) was added triethyl phosphonoacetate (1.55 mL, 7.72 mmol) at 0 oC under an argon atmosphere, then the mixture was stirred at the same temperature for 30 min. After cooling to -78 oC, to the solution was added a solution of the crude (2S,4S)-2-phenyl-1,3-dioxane-4-carbaldehyde (1.16 g, < 5.15 mmol) in THF (5 mL) at -78 oC, and the mixture was gradually warmed to rt and stirred for 1 h. The reaction mixture was poured into saturated aqueous NH4Cl solution, then the aqueous phase was extracted with hexane (x 3). The combined organic layers were washed with brine, dried over MgSO4, and concentrated in vacuo. The residue was purified by silica gel column chromatography (9% AcOEt in hexanes) to afford (E)-ethyl 3-((2S,4S)-2-phenyl-1,3-dioxan-4-yl)acrylate (6) (1.05 g, 78% yield in 2 steps) as a white solid.
6; white solid; mp 59.5-60.5 oC; [α]20D -25.5 (c 0.88, CHCl3); 1H NMR (300 MHz, CDCl3) δ 7.53-7.48 (m, 2H), 7.40-7.33(m, 3H), 6.96 (dd, J = 15.5, 4.1 Hz, 1H), 6.14 (dd, J = 15.5, 1.9 Hz, 1H), 5.58 (s, 1H), 4.54 (dtt, J = 11.6, 2.1, 2.1 Hz, 1H), 4.30 (ddd, J = 11.6, 4.8, 1.1 Hz, 1H), 4.20 (q, J = 7.1 Hz, 2H), 4.01 (ddd, J = 11.6, 11.6, 2.6 Hz, 1H), 1.98-1.83 (m,1H), 1.72-1.63 (m, 1H), 1.28 (t, J = 7.1 Hz, 3H); 13C NMR (75 MHz, CDCl3) δ 166.3, 145.9, 138.1, 128.9, 128.2(2C), 126.0(2C), 120.1, 101.1, 75.2, 66.7, 60.4, 30.6, 14.1; IR (KBr, cm-1) 2977, 2952, 2872, 1721, 1664, 1397, 1366, 1298, 1280, 1174, 1139, 1020, 976, 698; MS (EI) m/z 262 (M+, 3), 261(11), 217(2), 185(2), 140(12), 125(16), 106(40), 105(100), 97(28), 94(29), 77(42), 67(36), 55(20); (DART) m/z 263 ([M+H]+); HRMS-DART calcd. for C15H19O4 ([M+H]+) 263.1283, found 263.1306.
(E)-3-((2S,4S)-2-Phenyl-1,3-dioxan-4-yl)prop-2-en-1-ol
To a solution of (E)-ethyl 3-((2S,4S)-2-phenyl-1,3-dioxan-4-yl)acrylate (6) (10.0 g, 38.1 mmol) in THF (150 mL) was added dropwise diisobutylaluminum hydride (1.02 M in n-hexane, 112 mL, 114 mmol) over a period of 30 min at -78 oC under an argon atmosphere and the mixture was stirred at the same temperature for 15 min. The reaction mixture was quenched with saturated aqueous Na2SO4 solution, filtered through a pad of Celite® and the pad was washed with AcOEt. The filtrate was dried over MgSO4 and concentrated in vacuo. The residue was purified by silica gel column chromatography (hexane-AcOEt = 3:1 to 1:1, gradient) to afford (E)-3-((2S,4S)-2-phenyl-1,3-dioxan-4-yl)prop-2-en-1-ol (7.42 g, 88% yield) as a colorless oil.
allylic alcohol; colorless oil; [α]20D 12.1 (c 0.67, CHCl3); 1H NMR (300 MHz, CDCl3) δ 7.54-7.48 (m, 2H), 7.40-7.32(m, 3H), 5.97 (dtd, J = 15.5, 4.8, 1.0 Hz, 1H), 5.83 (dtd, J = 15.5, 3.4, 1.4 Hz, 1H), 5.58 (s, 1H), 4.45-4.37(m, 1H), 4.29 (ddd, J = 11.4, 5.2, 1.3 Hz, 1H), 4.21-4.13 (m, 2H), 4.06-3.95(m, 1H), 2.03-1.87(m, 1H), 1.66-1.56(m,1H); 13C NMR (75 MHz, CDCl3) δ 138.4, 130.73, 130.71, 128.8, 128.2(2C), 126.1(2C), 101.2, 76.8, 66.8, 62.9, 31.3; IR (neat, cm-1): 3402, 2857, 1398, 1371, 1106, 1025, 977, 756, 700; MS (EI) m/z: 220 (M+, 1), 219(4), 163(4), 134(11), 106(59), 105(100), 77(59), 67(20), 55(19); (DART) m/z 221 ([M+H]+); HRMS-DART calcd. for C13H17O3 ([M+H]+) 221.1178, found 221.1185.
(2S,4S)-2-Phenyl-4-((E)-3-(tetrahydro-2H-pyran-2-yloxy)prop-1-enyl)-1,3-dioxane (7)
To a solution of (E)-3-((2S,4S)-2-phenyl-1,3-dioxan-4-yl)prop-2-en-1-ol (6.63 g, 30.1 mmol) and p-toluenesulfonic acid (259 mg, 1.51 mmol) in CH2Cl2 (300 mL) was added 2H-dihydropyran (4.1 mL, 45.2 mmol) at 0 oC under an argon atmosphere. After stirring for 5min at the same temperature, the mixture was warmed to rt and stirred for 25 min. The reaction was quenched with saturated aqueous NaHCO3 solution and the aqueous phase was extracted with AcOEt/ hexane (1/1 v/v). The combined organic layers were washed with brine, dried over MgSO4, filtered and concentrated in vacuo. The residue was purified by silica gel column chromatography (hexane-AcOEt = 10:1 to 1:1, gradient) to afford (2S,4S)-2-phenyl-4-((E)-3-(tetrahydro-2H-pyran-2-yloxy)prop-1-enyl)-1,3-dioxane (7) (9.14 g, 99% yield) as a colorless oil.
7; colorless oil; 1H NMR (300 MHz, CDCl3) δ 7.55-7.47 (m, 2H), 7.41-7.29 (m, 3H), 5.98-5.80 (m, 2H), 5.57 (s, 1H), 4.64 (dd, J = 3.3, 3.3 Hz, 1H), 4.46-4.36 (m,1H), 4.34-4.22 (m, 2H), 4.08-3.74 (m, 2H), 3.87 (ddd, J = 11.2, 8.1, 3.4 Hz, 1H), 3.58-3.46 (m, 1H), 2.05-1.49 (m, 8H); IR (neat, cm-1) 2945, 2851, 1364, 1116, 1025, 980, 699; MS (EI) m/z 219(6), 203(11), 134(15), 106(58), 105(100), 85(71), 77(58), 55(40).
(3S,E)-3-(Benzyloxy)-6-(tetrahydro-2H-pyran-2-yloxy)hex-4-en-1-ol
To a solution of (2S,4S)-2-phenyl-4-((E)-3-(tetrahydro-2H-pyran-2-yloxy)prop-1-enyl)-1,3-dioxane (7) (8.54 g, 28.1 mmol) in toluene (128 mL) was added dropwise diisobutylaluminum hydride (1.01 M in n-hexane, 139 mL, 140 mmol) at 0 oC over a period of 10 min under an argon atmosphere. After stirring at 0 oC for 2 h, the mixture was warmed up to rt and stirred for 1 h. The reaction was quenched with saturated aqueous Na2SO4 solution, filtered through a pad of Celite® and the pad was washed with AcOEt (x 2). The filtrate was separated and dried over MgSO4 and concentrated in vacuo. The residue was purified by silica gel column chromatography (hexane-AcOEt = 3:1 to 1:1, gradient) to afford (3S,E)-3-(benzyloxy)-6-(tetrahydro-2H-pyran-2-yloxy)hex-4-en-1-ol (6.93 g, 81% yield) as a colorless oil.
alcohol; colorless oil; 1H NMR (300 MHz, CDCl3) δ 7.39-7.26(m, 5H), 5.83 (dt, J = 15.5, 5.3 Hz, 1H), 5.75-5.65 (m, 1H), 4.70-4.59 (m, 2H), 4.37 (d, J = 12.0 Hz, 1H), 4.33-4.24 (m, 1H), 4.12-4.00 (m, 2H), 3.93-3.83 (m, 1H), 3.83-3.68 (m, 2H), 3.57-3.48 (m,1H), 1.98-1.48 (m, 8H); IR (neat, cm-1) 3436, 2942, 2868, 1119, 1076, 1038; MS (EI) m/z 177(3), 113(13), 107(11), 91(100), 85(23), 55(13); (DART) m/z 307 ([M+H]+); HRMS-DART calcd. for C18H27O4 ([M+H]+) 307.1909, found 307.1909.
(3S,E)-3-(Benzyloxy)-6-(tetrahydro-2H-pyran-2-yloxy)hex-4-enal (3)
To a solution of (3S,E)-3-(benzyloxy)-6-(tetrahydro-2H-pyran-2-yloxy) hex-4-en-1-ol (7) (2.00 g, 6.53 mmol) in CH2Cl2 (60 mL) was added Dess-Martin periodinane (5.54 g, 13.1 mmol) at 0 oC. The mixture was warmed to rt and stirred for 1 h. After cooling, Dess-Martin periodinane (4.15 g, 9.78 mmol) was added to the mixture at 0 oC, then the mixture was warmed up to rt and stirred for 1 h. The reaction was quenched with saturated aqueous Na2S2O3 solution. To the mixture was added saturated aqueous NaHCO3 solution and the aqueous layer was extracted with AcOEt/ hexane (x 3). The combined organic layers were washed with brine, dried over MgSO4, filtered and concentrated in vacuo. The residue was purified by silica gel column chromatography (hexane-AcOEt = 5:1) to afford (3S,E)-3-(benzyloxy)-6-(tetrahydro-2H-pyran-2-yloxy)hex-4-enal (3) (1.94 g, 97% yield) as a colorless oil.
3; colorless oil; 1H NMR (300 MHz, CDCl3) δ 9.79-9.74 (m, 1H), 7.38-7.25 (m, 5H), 5.95-5.84(m, 1H), 5.78-5.65 (m, 1H), 4.70-4.55 (m,2H), 4.45-4.25 (m, 3H), 4.04 (ddd, J = 13.4, 5.5, 1.3 Hz, 1H), 3.94-3.82 (m, 1H), 3.60-3.46 (m, 1H), 2.76 (dddd, J = 16.5, 8.3, 2.8, 1.3 Hz, 1H), 2.63-2.52 (m, 1H), 1.92-1.48 (m, 6H); IR (neat, cm-1) 2943, 2868, 1726, 1119, 1077, 1026; MS (EI) m/z 256(2), 248(3), 219(2), 159(6), 131(5), 108(35), 107(39), 91(100), 85(67), 79(44), 77(33), 67(26), 55(27); (DART) m/z 305 ([M+H]+); HRMS-DART calcd. for C18H25O4 ([M+H]+) 305.1753, found 305.1764.
(3S)-3,5-Bis(benzyloxy)-2-vinyltetrahydrofuran (2)
To a solution of (3S,E)-3-(benzyloxy)-6-(tetrahydro-2H-pyran-2-yloxy)hex-4-enal (3) (200 mg, 0.66 mmol) and BnOH (213 mg, 1.97 mmol) in THF (33 mL) was added PdCl2(MeCN)2 (9 mg, 0.033 mmol) at rt under an argon atmosphere, then the mixture was stirred at the same temperature for 1 h. After stirring, the mixture was filtered through a pad of Florisil®, the pad was washed with AcOEt, and the filtrate was concentrated in vacuo. The residue was purified by silica gel column chromatography (hexane-AcOEt = 50:1 to 20:1, gradient) to afford (3S)-3,5-bis(benzyloxy)-2-vinyltetrahydrofuran (2) (113 mg, 55% yield) as a colorless oil.
2 (diastereomer mixture); colorless oil; 1H NMR (600 MHz, CDCl3) δ 7.38-7.24(m,10H), 6.10(ddd, J = 17.6, 10.3, 7.2 Hz, 0.1H), 5.94 (ddd, J = 17.2, 10.3, 7.0 Hz, 0.52H), 5.85 (ddd, J = 16.9, 10.4, 6.7 Hz, 0.38H), 5.38 (ddt, J = 17.2, 4.0, 1.3 Hz,0.9H), 5.44-5.30 (m, 0.3H), 5.32 (dd, J = 5.1, 2.2 Hz, 0.52H), 5.24 (dd, J = 5.7, 1.8 Hz, 0.38H), 5.21 (dt, J = 10.3, 1.3 Hz, 0.38H), 5.19 (dt, J = 10.3, 1.3 Hz, 0.52H), 4.77 (d, J = 11.7 Hz, 0.1H), 4.59-4.47 (m, 4H), 4.17-4.10 (m, 0.62H), 3.84 (ddd, J = 8.1, 5.7, 4.0 Hz, 0.38H), 2.36-2.27 (m, 1H), 2.24-2.20 (m, 0.1H), 2.18-2.13 (m, 0.52H), 2.10 (ddd, J = 15.9, 4.0, 1.8 Hz, 0.38H); MS (EI) m/z 254(3), 182(4), 163(5), 159(2), 108(9), 107(7), 91(100), 79(12), 77(8), 65(10), 51(4); (DART) m/z 311 ([M+H]+); HRMS-DART calcd. for C20H23O3 ([M+H]+) 311.1647, found 311.1675.
((3S)-3,5-Bis(benzyloxy)tetrahydrofuran-2-yl)methanol (8)
To a solution of (3S)-3,5-bis(benzyloxy)-2-vinyltetrahydrofuran (2) (diastereomer mixture; 300 mg, 0.97 mmol) in 1,4-dioxane (9 mL) and H2O (5 mL) was added 2,6-lutidine (207 mg, 1.94 mmol), NaIO4 (414 mg, 1.94 mmol) and OsO4 (0.05 mol/L in tert-BuOH, 0.4 mL, 0.02 mmol), then the mixture was stirred at rt for 16 h. The mixture was diluted with AcOEt and H2O, the aqueous phase was extracted with AcOEt (x 1). The combined organic layers were washed with saturated aqueous Na2S2O3 solution and brine, dried over MgSO4, filtered and concentrated in vacuo. The residue was diluted with MeOH (5 mL), to this solution was added NaBH4 (73 mg, 1.94 mmol) at rt. After stirring at the same temperature for 30 min, the mixture was quenched with saturated NH4Cl solution and concentrated in vacuo. The residue was diluted with AcOEt and H2O, then the organic layer was separated and the aqueous layer was extracted with AcOEt (x1). The combined organic layers were washed with 1 N HCl and brine, dried over MgSO4, filtered and concentrated in vacuo. The residue was purified by silica gel column chromatography (hexane-AcOEt = 3:1) to afford ((3S)-3,5-bis(benzyloxy)tetrahydrofuran-2-yl)methanol (8) (304 mg, 53% yield in 2 steps). Three diastereomers were separated in this step.
((2R,3S,5R)-3,5-Bis(benzyloxy)tetrahydrofuran-2-yl)methanol (8a); colorless oil; [α]20D -65.6 (c 1.60, CHCl3); 1H NMR (300 MHz, CDCl3) δ 7.38-7.26 (m, 10H), 5.36 (dd, J = 5.9, 2.1 Hz, 1H), 4.76 (d, J = 11.7 Hz, 1H), 4.53 (d, J = 11.7 Hz, 1H), 4.49 (s, 2H), 4.35-4.26 (m, 2H), 3.76 (dd, J = 12.0, 2.7 Hz, 1H), 3.64 (ddd, J = 12.0, 8.5, 3.9 Hz, 1H), 2.43 (ddd, J = 14.1, 6.9, 2.0 Hz, 1H), 2.23 (dt, J = 14.1, 5.3 Hz, 1H); 13C NMR (75 MHz, CDCl3) δ 137.7, 137.1, 128.53(2C), 128.46(2C), 128.0(2C), 127.9, 127.8, 127.7(2C), 104.0, 85.7, 79.0, 71.7, 70.0, 64.1, 40.2; IR (neat, cm-1) 3447, 2925, 2872, 1455, 1361, 1104, 1043, 1026, 698, 607; MS (EI) m/z 254(1), 207(7), 181(4), 163(2), 146(2), 108(32), 107(25), 91(100), 79(41), 77(26), 65(12), 51(12); (DART) m/z 315 ([M+H]+); HRMS-DART calcd. for C19H23O4 ([M+H]+) 315.1596, found 315.1608.
((2R,3S,5S)-3,5-Bis(benzyloxy)tetrahydrofuran-2-yl)methanol (8b); colorless oil; [α]20D -34.7 (c 0.33, CHCl3); 1H NMR (300 MHz, CDCl3) δ 7.39-7.27 (m, 10H), 5.39 (dd, J = 5.2, 1.9 Hz, 1H), 4.73 (d, J = 11.9 Hz, 1H), 4.59 (d, J = 11.7 Hz, 1H), 4.51-4.41 (m, 1H), 4.49 (d, J = 11.9 Hz, 1H), 4.42 (d, J = 11.7 Hz, 1H), 4.20 (dt, J = 5.8, 4.3 Hz, 1H), 4.00-3.85 (m, 2H), 2.29 (ddd, J = 13.4, 6.7, 1.9 Hz, 1H), 2.19 (ddd, J = 13.4, 5.2, 5.2 Hz, 1H); 13C NMR (75 MHz, CDCl3) δ 137.9, 137.4, 128.6(2C), 128.4(2C), 128.00, 127.97(3C), 127.7, 127.6, 102.2, 79.4, 79.0, 71.8, 69.3, 61.8, 39.6; IR (neat, cm-1) 3444, 2921, 2873, 1061, 1026, 737, 698; MS (EI) m/z 254(2), 207(3), 206(3), 182(3), 181(4),163(4), 149(3), 146(2), 108(34), 107(31), 91(100), 79(39), 77(29), 57(14); (DART) m/z 315 ([M+H]+); HRMS-DART calcd. for C19H23O4 ([M+H]+) 315.1596, found 315.1607.
((2S,3S,5R)-3,5-Bis(benzyloxy)tetrahydrofuran-2-yl)methanol (8c); colorless oil; [α]20D 162.4 (c 1.70, CHCl3); 1H NMR (300 MHz, CDCl3) δ 7.40-7.25 (m, 10H), 5.24 (dd, J = 5.3, 1.5 Hz, 1H), 4.81 (d, J = 12.4 Hz, 1H), 4.60 (d, J = 12.0 Hz, 1H), 4.54 (d, J = 12.4 Hz, 1H), 4.49 (d, J = 12.0 Hz, 1H), 4.20 (dd, J = 8.1, 4.0 Hz, 1H), 4.08-4.00 (m, 1H), 3.82 (dd, J = 11.9, 2.9 Hz, 1H), 3.61 (dd, J = 11.9, 2.9 Hz, 1H), 2.26 (ddd, J = 13.4, 8.3, 5.4 Hz, 1H), 2.12 (ddd, J = 14.1, 3.4, 1.7 Hz, 1H); 13C NMR (75 MHz, CDCl3) δ 138.0(2C), 128.4(2C), 128.3(2C), 127.8(2C), 127.7(2C), 127.5(2C), 102.7, 82.9, 78.1, 71.7, 68.9, 62.6, 39.1; IR (neat, cm-1) 3445, 2920, 2872, 1454, 1360, 1207, 1113, 1043, 1027, 737, 698; MS (EI) m/z 207(11), 206(2), 10(21), 107(19), 91(100), 79(26), 77(18), 65(11), 51(10); (DART) m/z 315 ([M+H]+); HRMS-DART calcd. for C19H23O4 ([M+H]+) 315.1596, found 315.1610.
2-Deoxy-D-ribose
To a solution of 8a (16 mg, 0.051 mmol) in EtOH (1 mL) was added Pd/C (2 mg) at rt, then the reaction vessel was evacuated and back-filled with H2 (5x). After stirring for 58 h at rt, the mixture was filtered over a pad of Celite®, which was washed with MeOH. The filtrate was concentrated in vacuo. The crude material was purified by silica gel column chromatography (MeOH-CHCl3 = 1:3) to afford 2-deoxy-D-ribose (6.6 mg, 97% yield) as a colorless amorphous solid.
2-Deoxy-D-ribose; colorless amorphous solid; [α]20D -38.6 (c 0.33, MeOH); 1H NMR (300 MHz, CD3OD) δ difficult to assign the spectra; 13C NMR (75 MHz, CD3OD) the equilibrium mixture of pyranose and furanose form and those epimer at C1; δ 99.5, 99.4, 95.2, 93.1, 87.9, 86.7, 72.8, 72.5, 69.4, 69.2, 68.3, 66.6, 66.2, 64.5, 64.3, 63.2, 43.5, 43.1, 37.3, 36.6.
ACKNOWLEDGEMENTS
This study was financially supported by a Grant-in-Aid for Scientific Research from the Ministry of Education, Culture, Sports, Science, and Technology of Japan.
References
1. Y. Ding, Chem. Lett., 2006, 35, 952; CrossRef M. Zhong, I. Nowak, and M. J. Robins, J. Org. Chem., 2006, 71, 7773; CrossRef C. Genu-Dellac, G. Gosselin, and J.-L. Imbach, Tetrahedron Lett., 1991, 32, 79. CrossRef
2. Y. E. Raifeld, A. Nikitenko, and B. M. Arshava, Tetrahedron, 1993, 49, 2509; CrossRef M. A. Findeis and G. M. Whitesides, J. Org. Chem., 1987, 52, 2838; CrossRef Y. Yamamoto, M. Kirihata, I. Ichimoto, and H. Ueda, Agric. Biol. Chem., 1985, 49, 1435; CrossRef T. Harada and T. Mukaiyama, Chem. Lett., 1981, 1109; CrossRef L. Vargha and J. Kuszmann, Chem. Ber., 1963, 96, 411; CrossRef G. Rembarz, Chem. Ber., 1962, 95, 1565; CrossRef E. F. Recondo and H. Rinderknecht, Helv. Chim. Acta, 1960, 43, 1653; CrossRef J. C. Sowden, J. Am. Chem. Soc., 1954, 76, 3541; CrossRef Y. Matsushima and Y. Imanaga, Nature, 1953, 171, 475. CrossRef
3. Y. Hirai, T. Terada, Y. Amemiya, and T. Momose, Tetrahedron Lett., 1992, 33, 7893; CrossRef Y. Hirai and M. Nagatsu, Chem. Lett., 1994, 21; CrossRef Y. Hirai, K. Shibuya, Y. Fukuda, H. Yokoyama, and S. Yamaguchi, Chem. Lett., 1997, 221; CrossRef Y. Hirai, J. Watanabe, T. Nozaki, H. Yokoyama, and S. Yamaguchi, J. Org. Chem., 1997, 62, 776; CrossRef H. Yokoyama, K. Otaya, S. Yamaguchi, and Y. Hirai, Tetrahedron Lett., 1998, 39, 5971; CrossRef H. Yokoyama, K. Otaya, H. Kobayashi, M. Miyazawa, S. Yamaguchi, and Y. Hirai, Org. Lett., 2000, 2, 2427; CrossRef M. Miyazawa, M. Narantsetseg, H. Yokoyama, S. Yamaguchi, and Y. Hirai, Heterocycles, 2004, 63, 1017. CrossRef
4. M. Miyazawa, Y. Hirose, M. Narantsetseg, H. Yokoyama, S. Yamaguchi, and Y. Hirai, Tetrahedron Lett., 2004, 45, 2883. CrossRef
5. T. Tsuri and S. Kamata, Tetrahedron Lett., 1985, 26, 5195; CrossRef S. Hanessian, A. Ugolini, and M. Therien, J. Org. Chem., 1983, 48, 4427. CrossRef
6. D. B. Dess and J. C. Martin, J. Am. Chem. Soc., 1991, 113, 7277. CrossRef