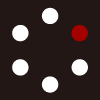
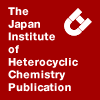
HETEROCYCLES
An International Journal for Reviews and Communications in Heterocyclic ChemistryWeb Edition ISSN: 1881-0942
Published online by The Japan Institute of Heterocyclic Chemistry
e-Journal
Full Text HTML
Received, 28th June, 2012, Accepted, 17th July, 2012, Published online, 25th July, 2012.
DOI: 10.3987/COM-12-12536
■ Sulfur-Containing Pyridylazulenes: Synthesis and Chromogenic Behaviors for Heavy Metal Ions
Shigeharu Wakabayashi,* Rina Yamaoka, Emi Matsumoto, Mitsue Nishiguchi, Miki Ishiura, Misaki Tsuji, and Makoto Shimizu
Department of Clinical Nutrition, Faculty of Health Science, Suzuka University of Medical Science, Suzuka, Mie 510-0293, Japan
Abstract
A facile method for the synthesis of azulene derivatives bearing 2-pyridyl group at the 1-position, and thio or methylthio group at the 2-position is described. The color and spectral changes that occur upon the addition of heavy metal ions were examined. It was found that the sulfur functional group in pyridylazulene did not effectively participate in the improvement of color selectivity for heavy metal ions, whereas the sensitivity for Hg2+ ion seemed to be slightly improved.INTRODUCTION
Although it is well known that the addition of a protic acid or metal ions to azulene derivatives causes a color change,1 the development of more sophisticated systems which also undergo a color change would be of great significance to further understand azulenic chromophores. Recently, we reported the synthesis of pyridylazulenes (for example, 1), and investigated in detail the color and spectral changes that occurred upon the addition of an acid or metal ions.2 The color changed from blue to red upon the addition of trifluoroacetic acid or soft heavy metal ions such as Hg2+ and Pb2+, depending on the substitution patterns of the pyridyl group on the azulene skeleton.
Selective response toward metal ions or colorimetric sensing of Hg2+ ion is currently an important research area in molecular recognition chemistry, as exemplified with azacrown ethers and azocalix[4]arene, etc.3 In the course of our studies on high sensing of Hg2+ ion in azulene chemistry,4 we designed compounds 2 and 3, having thio and methylthio groups at the 2-position of the azulene skeleton, respectively, since Hg2+ ion has high affinity for soft donors such as sulfur. It is of interest to investigate the influence of the sulfur functional group on the selectivity and sensitivity toward Hg2+ ion. Here, we describe the protocol for their synthesis, and the chromogenic behaviors of these compounds for heavy metal ions, especially Hg2+ ion. The structural studies of the product are also presented.
RESULTS AND DISCUSSION
The syntheses of pyridylazulenes 2 and 3 are summarized in Scheme 1, showing the use of 2-azulenethiol (4)5 as a common starting material. In order to protect the thiol (-SH) group, 4 was reacted with dimethylcarbamoyl chloride in the presence of KOH in toluene at 120 ºC,6 providing thiocarbamate 55 in 72% yield. Subsequent iodination with N-iodosuccinimide (NIS) occurred smoothly in CHCl3 at room temperature to afford iodide 6, which was treated with 2-(trimethylstannyl)pyridine in the presence of Pd(PPh3)4, CuI, and CsF in DMF at 50 ºC7 to give the pyridyl derivative 7 in 60% yield. Finally, the attempted hydrolysis of 7 with KOH in EtOH–H2O at 50 ºC or hydrazine in MeOH at 80 ºC8 gave 2 in only 27%, 5% yield, respectively; however, by treatment with Ba(OH)2 in MeOH–H2O at room temperature, 7 was easily deprotected to give 2 as a green solid in 71% yield.9 In a similar manner, 2-methylthioazulene (8), prepared from 4 according to the literature,5 was treated with NIS in CHCl3, followed by 2-(trimethylstannyl)pyridine under Stille coupling conditions,7 forming 3 as a violet oil in 61% yield.
The structures of 2 and 3 were established by NMR, IR, and MS analyses, and the compounds were stable and showed no deterioration after storage for 1 year at –20 ºC.
We studied changes in the spectral properties and colors of 2 and 3 upon addition of heavy metal ions. The coordination of soft heavy metal ions onto the pyridine moiety was expected to enhance its electron-withdrawing ability and influence the π-conjugate system of azulene.4 The individual addition of heavy metal ions (Ag+, Hg2+, Pb2+, Cu2+, Cr3+) as the perchlorate to 2 in acetone produced red or reddish brown color changes.10 The individual addition of Hg2+, Pb2+, Cr3+ ions to 3 produced reddish brown color changes, although Ag+ and Cu2+ ions caused little color change and a dark brown color change, respectively. Thus, 2 and 3 did not exhibit the Hg2+ selectivity as in the case of 1. The representative spectral changes are shown in Figure 1, revealing an isosbestic point at 565 nm. These observations suggest that the sulfur functional group in 2 and 3 did not effectively participate in the improvement of color selectivity for heavy metal ions. It is conceivable that some metal ions, which produced color changes, bind to the pyridine moiety, regardless of the sulfur functional group.
Interestingly, upon careful addition of 0.2 equiv of Hg(ClO4)2, the color of 2 and 3 changed from violet to red. Compared with 1, which required 2 equiv of Hg2+ ion for color change,2 2 and 3 seem to show slightly improved sensitivity for Hg2+ ion. With the concentration of pyridylazulene employed in our studies, Hg2+ ion concentrations as low as 10-4 M could be detected, i.e, concentrations in the ppm range.
To gain insight into the interaction of pyridylazulene 3 with Hg2+ ion, 1H NMR, ESI-TOF-MS, and Job’s plot analyses were performed. In the 1H NMR spectrum of the complex formed in the reaction of 3 and 1 equiv of Hg(ClO4)2 in acetone-d6, the Me protons of the SMe group were shifted downfield by 0.19 ppm (3, δ 2.64; 3•Hg2+, δ 2.83), in addition to the downfield shifts of the aromatic protons. ESI-TOF-MS of the complex in acetone gave a charged peak at m/z 510, corresponding to [3•Hg•acetone–H]+ (Figure 2). The observed isotopic and theoretical distributions were in close agreement. These data suggest that 3 strongly interacts with Hg2+ ion in acetone, in which both the sulfur donor atom and nitrogen atom of pyridine moiety should coordinate to Hg2+ ion. Further support of the 1:1 binding ratio comes from a Job’s plot experiment,11 where the absorption of the complex at 514 nm was plotted against the molar fraction of 3 at an invariant total concentration. As a result, the concentration of the 3•Hg2+ complex approached a maximum when the molar fraction of [3] / ([3] + [Hg2+]) was about 0.5, therefore 1:1 association constant was estimated on the basis of Benesi-Hilderbrand plot12 to give 330 M-1.13
In summary, a synthetic method for pyridylazulenes bearing a sulfur functional group has been developed. It is worth noting that the deprotection of thiocarbamate moiety in 7 was improved by the use of Ba(OH)2.9 The color selectivity for Hg2+ ion in 2 and 3 did not improved as compared with 1, although an S-coordinated Hg2+-complex would be easily formed. It can be seen that the coordination bond of pyridine moiety with Hg2+ ion, which is responsible for a color change, was little subject to the influence of the sulfur functional group. The sensitivity for Hg2+ ion seemed to be slightly improved as compared with 1.2 Further study toward high sensing of heavy metal ions in azulene chemistry is in progress.
EXPERIMENTAL
Melting points were determined on a Shibata MEL-270 melting point apparatus and are uncorrected. 1H and 13C NMR spectra were recorded in CDCl3 on a Varian 400-MR spectrometer and referenced to internal TMS or solvent signals. IR spectra were measured with a Shimadzu IRAffinity-1 spectrophotometer. UV-Vis spectra were obtained on Shimadzu UV-2200 and Hitachi U-3000 spectrophotometer. Electron impact mass spectra (EIMS) and high resolution mass spectra (HRMS) were measured at a voltage of 70 eV on a Jeol JMS T100GCV. Electrospray ionization mass spectrum (ESI-TOF-MS) was recorded on a Brucker Daltonics microtof-SZ. All reagents and solvents were purchased from commercial sources and used as received. Most reactions were carried out in serum-capped, oven-dried, and argon-purged flasks. TLC analyses were conducted on Merck Kieselgel 60 F254-precoated plates with detection by UV light.
2-Azulenyl N,N-dimethylthiocarbamate (5)
To a soln of 2-azulenethiol (4) (330 mg, 2.06 mmol) in toluene (10 mL) were added dimethylcarbamoyl chloride (1.40 mL, 15.2 mmol) and KOH (139 mg, 2.48 mmol) and the mixture was stirred at 120 ºC for 20 h. The reaction mixture was poured into H2O (30 mL) and extracted with ether (60 mL). The organic layer was washed with brine (2 × 10 mL), dried over MgSO4, and concentrated. The crude product was subjected to silica gel column chromatography [silica gel (10 g), hexane, then benzene–hexane (1:1)] to afford 5 (341 mg, 72%) as a blue solid. mp 154–155 ºC. (Lit.5 blue needles, mp 156 ºC) 1H NMR (400 MHz, CDCl3) δ 3.10 (bs, 3H), 3.15 (bs, 3H), 7.18 (t, J = 9.6 Hz, 2H), 7.55 (s, 2H), 7.56 (t, J = 9.6 Hz, 2H), 8.25 (d, J = 9.7 Hz, 2H). 13C NMR (100 MHz, CDCl3) δ 37.0, 120.3, 123.8, 128.3, 136.1, 137.3, 139.7,165.6. IR (KBr): 3447, 1667, 1364, 1094 cm-1. MS (EI, 70 eV) m/z 231 (M+, 42), 159 (M+–CONMe2, 20). HRMS (EI): m/z calcd for C13H13NOS 231.0718, found 231.0716.
1-Iodoazulen-2-yl N,N-dimethylthiocarbamate (6)
To a soln of 5 (340 mg, 1.472 mmol) in CHCl3 (20 mL) was added NIS (337 mg, 1.498 mmol) and the mixture was stirred at rt for 30 min. The mixture was directly subjected to column chromatography using alumina (25 g) and a 1:5 mixture of EtOAc and hexane as eluent to afford 6 (574 mg, 100%) as a blue oil. 1H NMR (400 MHz, CDCl3) δ 3.08 (bs, 3H), 3.19 (bs, 3H), 7.21 (t, J = 9.8 Hz, 1H), 7.28 (t, J = 9.8 Hz, 1H), 7.59 (t, J = 9.8 Hz, 1H), 7.86 (s, 1H), 8.18 (d, J = 9.6 Hz, 1H), 8.25 (d, J = 9.8 Hz, 1H).
13C NMR (100 MHz, CDCl3) δ 37.1, 122.1, 124.6, 124.7, 128.3, 136.3, 138.2, 138.6, 139.6, 140.8, 141.6, 164.5. IR (neat): 2926, 1661, 1574, 1393, 1360, 1258, 1094 cm-1.
1-(2-Pyridyl)azulen-2-yl N,N-dimethylthiocarbamate (7)
A mixture of 6 (410 mg, 1.15 mmol), Pd(PPh3)4 (88 mg, 0.0762 mmol), CuI (45 mg, 0.240 mmol), CsF (431 mg, 2.84 mmol), and 2-(trimethylstannyl)pyridine (490 mg, 2.03 mmol) in DMF (10 mL) was stirred at 50 ºC for 3 h. After this time, the reaction mixture was poured into H2O (30 mL) and extracted with EtOAc (80 mL). The organic layer was washed with brine (2 × 10 mL), dried over MgSO4, and concentrated. The crude product was subjected to silica gel column chromatography [silica gel (18 g), benzene–hexane (1:1), then benzene, then benzene–ether (9:1)] to afford 7 (213mg, 60%) as a blue oil.
1H NMR (400 MHz, CDCl3) δ 3.07 (bs, 3H), 3.12 (bs, 3H), 7.22 (t, J = 10.0 Hz, 2H), 7.24–7.27 (m, 1H), 7.59 (t, J = 9.8 Hz, 1H), 7.65 (dt, J = 1.0, 8.0 Hz, 1H), 7.796 (td, J = 7.6, 1.9 Hz, 1H), 7.802 (s, 1H), 8.32 (d, J = 9.4 Hz, 1H), 8.57 (d, J = 9.6 Hz, 1H), 8.80 (dm, J = 5.7 Hz, 1H). 13C NMR (100 MHz, CDCl3) δ 37.0, 121.1, 121.8, 124.5, 125.1, 126.2, 128.3, 129.9, 135.8, 136.1, 136.96, 137.04, 138.2, 139.9, 149.4, 154.7, 165.8. IR (neat): 3032, 2928, 1667, 1585, 1572, 1485, 1404, 1360, 1258, 1096 cm-1. MS (EI, 70 eV) m/z 308 (M+, 30), 236 (M+–HSCH2CON(CH3)2, 100). HRMS (EI): m/z calcd for C18H16N2OS 308.0983, found 308.0978.
1-(2-Pyridyl)azulene-2-thiol (2)
To a soln of 7 (189 mg, 0.614 mmol) in MeOH (13.5 mL)–H2O (2.7 mL) was added Ba(OH)2•8H2O (571 mg, 1.810 mmol) and the mixture was stirred at rt for 5.5 h. To the reaction mixture was added 2N HCl (3 mL) and pH was adjusted to 3–4. The reaction mixture was poured into H2O (20 mL) and extracted with benzene (30 mL, 2 × 15 mL). The combined organic layer was washed with brine (2 × 10 mL), dried over MgSO4, and concentrated. The crude product was subjected to silica gel column chromatography [silica gel (8 g), benzene, then benzene–ether (9:1)] to afford 2 (103 mg, 71%) as a green solid. mp 78–80 ºC. 1H NMR (400 MHz, CDCl3) δ 7.14 (t, J = 9.8 Hz, 1H), 7.21–7.26 (m, 2H), 7.49 (t, J = 10.0 Hz, 1H), 7.67 (s, 1H), 7.79–7.86 (m, 2H), 8.04 (d, J = 9.4 Hz, 1H), 8.59 (d, J = 9.8 Hz, 1H), 8.84 (dt, J = 1.3, 4.5 Hz, 1H). 13C NMR (100 MHz, CDCl3) δ 115.9, 120.8, 124.4, 125.2, 125.7, 125.8, 128.3, 132.9, 134.8, 136.3, 136.6, 137.7, 140.5, 148.4, 149.5, 154.3. IR (KBr): 3447, 3012, 1584, 1481, 1402, 1387 cm-1. MS (EI, 70 eV) m/z 237 (M+, 88), 236 (M+–1, 89), 204 (M+–SH, 100). HRMS (EI): m/z calcd for C15H11NS 237.0612, found 237.0612. UV/Vis (acetone) λmax (ε) = 328 (30200), 402 (7300, sh), 560 (260), 600 nm (220, sh).
2-Methylthio-1-(2-pyridyl)azulene (3)
To a soln of 2-methylthioazulene (8) (290 mg, 1.67 mmol) in CHCl3 (15 mL) was added NIS (398 mg, 1.77 mmol) and the mixture was stirred at rt for 1 h. The mixture was directly subjected to column chromatography [alumina (20 g), hexane] to afford iodide 9 (505 mg, 100%) as a blue oil. To this were added Pd(PPh3)4 (138 mg, 0.119 mmol), CsF (605 mg, 3.983 mmol), CuI (65 mg, 0.341 mmol), and a soln of 2-(trimethylstannyl)pyridine (744 mg, 3.079 mmol) in DMF (12 mL). After 2.5 h of stirring at 50 ºC, the mixture was poured into H2O (30 mL) and extracted with EtOAc (60 mL, 2 × 20 mL). The combined organic layer was washed with brine (2 × 10 mL) and dried over MgSO4. The crude product was subjected to silica gel column chromatography [silica gel (22 g), EtOAc–hexane (1:20), then (1:8), then (1:5)] to afford 3 (253 mg, 61%) as a violet oil. 1H NMR (400 MHz, CDCl3) δ 2.64 (s, 3H), 7.17–7.24 (m, 4H), 7.47 (t, J = 9.8 Hz, 1H), 7.70 (dt, J = 8.0, 1.0 Hz, 1H), 7.77 ( td, J = 7.8, 2.0 Hz, 1H), 8.12 (d, J = 9.4 Hz, 1H), 8.54 (d, J = 10.0 Hz, 1H), 8.79 (dm, J = 4.9 Hz, 1H). 13C NMR (100 MHz, CDCl3) δ 15.8, 112.4, 120.8, 124.8, 125.1, 125.4, 125.7, 132.1, 132.8, 135.4, 136.0, 137.4, 141.1, 149.6, 149.6, 154.8. IR (neat): 3049, 3017, 2920, 1709, 1585, 1562, 1483, 1437, 1408, 1391 cm-1. MS (EI, 70 eV) m/z 251 (M+, 62), 236 (M+–Me, 100), 218 (73). HRMS (EI): m/z calcd for C16H13NS 251.0769, found 251.0757. UV/Vis (acetone) λmax (ε) = 331 (39100), 388 (7200), 405 (7200), 554 (240), 590 nm (210, sh).
General procedures for the titration experiments by UV/vis.
Screw-capped quartz cells were used in order to prevent the volatilization of solvent and to mix up the samples. To a soln of azulene derivative in acetone (3.4 × 10-3 M, 3 mL) was added a 0.761 M soln of Hg(ClO4)2 in acetone with a pipetman. After mixing at r.t., the changes of color were checked by the naked eye and UV/vis spectra were recorded.
ACKNOWLEDGEMENTS
We are grateful to Professors S. Ito of Hirosaki University, K. Hirose of Osaka University, and W.-S. Chung of National Chiao Tung University for their valuable suggestions. We also acknowledge Professors K. Tatsumi, Y. Ohki, and Dr. K. Oyama of Nagoya University for their help in MS spectroscopy.
References
1. For acid, see: (a) Pl. A. Plattner, E. Heilbronner, and S. Weber, Helv. Chim. Acta, 1952, 35, 1036; (b) Chopard-dit-Jean and L. H. Heilbronner, Helv. Chim. Acta, 1952, 35, 2170; CrossRef (c) H. M. Frey, J. Chem. Phys., 1956, 25, 600; CrossRef (d) S. S. Danyluk and W. G. Schneider, J. Am. Chem. Soc., 1960, 82, 997; CrossRef (e) E. C. Kirby and D. H. Reid, J. Chem. Soc., 1960, 494; CrossRef (f) K. Hafner and H. Pelster, Angew. Chem., 1960, 72, 781; (g) R. S. H. Liu, R. S. Muthyala, X.-S. Wang, A. E. Asato, P. Wang, and C. Ye, Org. Lett., 2000, 2, 269; CrossRef (h) R. S. H. Liu, J. Chem. Edu., 2002, 79, 183; CrossRef (i) S. V. Shevyakov, H. Li, R. Muthyala, A. E. Asato, J. C. Croney, D. M. Jameson, and R. S. H. Liu, J. Phys. Chem. A, 2003, 107, 3295; CrossRef For metal ions, see: (j) H.-G. Löhr, F. Vögtle, W. Schuh, and H. Puff, Chem. Ber., 1984, 117, 2839; CrossRef (k) H.-G. Löhr and F. Vögtle, Chem. Ber., 1985, 118, 905; CrossRef (l) H.-G. Löhr and F. Vögtle, Acc. Chem. Res., 1985, 18, 65. CrossRef
2. S. Wakabayashi, Y. Kato, K. Mochizuki, R. Suzuki, M. Matsumoto, Y. Sugihara, and M. Shimizu, J. Org. Chem., 2007, 72, 744. CrossRef
3. (a) K. Rurack, M. Kollmannsberger, U. Resch-Genger, and J. Daub, J. Am. Chem. Soc., 2000, 122, 968; CrossRef (b) L. Prodi, C. Bargossi, M. Montalti, N. Zaccheroni, N. Su, J. S. Bradshaw, R. M. Izatt, and P. B. Savage, J. Am. Chem. Soc., 2000, 122, 6769; CrossRef (c) F. Sancenon, R. Martinez-Manez, and J. Soto, Tetrahedron Lett., 2001, 42, 4321; CrossRef (d) M. J. Choi, M. Y. Kim, and S.-K. Chang, Chem. Commun., 2001, 1664; CrossRef (e) A. B. Descalzo, R. Martinez-Manez, R. Radeglia, K. Rurack, and J. Soto, J. Am. Chem. Soc., 2003, 125, 3418; CrossRef (f) E. M. Nolan and S. J. Lippard, J. Am. Chem. Soc., 2003, 125, 14270; CrossRef (g) T.-L. Kao, C.-C. Wang, Y.-T. Pan, Y.-J. Shiao, J.-Y. Yen, C.-M. Shu, G.-H. Lee, S.-M. Peng, and W.-S. Chung, J. Org. Chem., 2005, 70, 2912; CrossRef (h) I.-T. Ho, G.-H. Lee, and W.-S. Chung, J. Org. Chem., 2007, 72, 2434; CrossRef (i) A. F. D. de Namor, I. Abbas, and H. H. Hammud, J. Phys. Chem. B, 2007, 111, 3098; CrossRef (j) A. F. D. de Namor and I. Abbas, J. Phy. Chem. B, 2007, 111, 5803; CrossRef (k) J. Huang, Y. Xu, and X. Qian, J. Org. Chem., 2009, 74, 2167. CrossRef
4. S. Wakabayashi, R. Uriu, T. Asakura, C. Akamatsu, and Y. Sugihara, Heterocycles, 2008, 75, 383. CrossRef
5. (a) T. Asao, S. Ito, and N. Morita, Tetrahedron Lett., 1989, 30, 6345; CrossRef (b) T. Asao, Pure & Appl. Chem., 1990, 62, 507. CrossRef
6. K. S. Jeong and H. K. Oh, Bull. Korean Chem. Soc., 2007, 28, 485. CrossRef
7. S. P. H. Mee, V. Lee, and J. E. Baldwin, Angew. Chem. Int. Ed., 2004, 43, 1132. CrossRef
8. (a) K. Krowicki, Polish J. Chem., 1979, 53, 503; (b) L. L. Whitfield, Jr., and E. P. Papadopoulos, Synthesis, 1985, 423. CrossRef
9. Regarding the improvement of yield in deprotection reaction, it
is possible that hydrolysis is disturbed by the back reaction as
shown in A using monovalent metal ion such as K+, whereas the
divalent anion, which might be slightly formed with Ba(OH)2,
retard the back reaction, therefore resulting in the easy
elimination of RS- as shown in B. Furthermore, the
nucleophilicity of OH- could be enhanced since Ba2+ ion has the relatively large ionic radius.
10. In our previous work, we used the nitrate salt and MeOH-H2O as well as the perchlorate and acetone. However, since we could not observe the special effect on color and spectral changes, we also used perchlorate salt and acetone as solvent in the present work.
11. K. Hirose, J. Inclusion Phenom. Macrocyclic Chem., 2001, 39, 193. CrossRef
12. H. A. Benesi and J. H. Hildebrand, J. Am. Chem. Soc., 1949, 71, 2703. CrossRef
13. Unfortunately, most spectral changes did not have the isosbestic point, probably because of the formation of complex mixture. Among the complexes investigated in the present work, the complex of 3 with Pb2+ ion also afforded the 1:1 binding ratio from the Job’s plot and 220 M-1 as the association constant.