|
 |
Year : 2015 | Volume
: 9
| Issue : 2 | Page : 33-37 |
|
|
|
|
ORIGINAL ARTICLE A quantitative analysis of the effect of baseplate and glenosphere position on deltoid lengthening in reverse total shoulder arthroplasty
Jonathan Wright1, Christopher Potts1, Mark P Smyth1, Lisa Ferrara2, John W Sperling3, Thomas W Throckmorton1
1 Department of Orthopaedic Surgery and Biomedical Engineering, University of Tennessee, Campbell Clinic, Memphis, TN 38104, USA 2 Director of Ortho Kinetic Technologies LLC, Shallotte, NC 28470, USA 3 Department of Orthopaedic Surgery, Mayo Clinic, Rochester, MN 55905, USA
Correspondence Address: Thomas W Throckmorton 1211 Union Avenue, Suite 510, Memphis, TN 38104 USA
 Source of Support: None, Conflict of Interest: None
DOI: 10.4103/0973-6042.154752
 |
|
|
|
Date of Web Publication | 8-Apr-2015 |
Abstract | | |
Context: Optimizing deltoid tension is important to achieve maximal function after reverse total shoulder arthroplasty (RTSA), but the effects of baseplate and glenosphere positions on deltoid tension have not been quantified. Aims: To quantify deltoid elongation and elongation to failure under physiologic loads with three baseplate-glenosphere configurations with increasing inferior offset. Settings and Design: Cadaver biomechanical study. Materials and Methods: Twenty-four cadaver shoulders were divided into three groups. The starting point for baseplate insertion in Group 1 was the center of the glenoid, with glenospheres placed in minimal inferior offset (0.5 mm). Groups 2 and 3 baseplates were placed 2 mm inferior to the center point and glenospheres in minimal (2.5 mm) offset (Group 2) or maximal (4.5 mm) offset (Group 3). Tensile testing was done to quantify deltoid elongation and evaluate failure. Statistical Analysis Used: A one-way analysis of variance was performed to detect statistically significant differences among treatment groups. A post-hoc Neuman-Keul's comparison was conducted to perform discrete comparisons among treatment groups. Results: Deltoid elongation after loading decreased with increasing inferior offset of >2.5 mm. No significant difference in deltoid yield load was found among groups. The percent of elongation was decreased significantly between groups 2 and 3. Deltoid displacement at failure decreased from 33.3 mm for Group 2-17.3 mm for Group 3. 16 of the 24 specimens (67%) failed by anterior deltoid detachment from the acromion. Conclusions: Increasing inferior offset in RTSA constructs appears to increase stretch forces on the deltoid, resulting in a diminished ability of the deltoid to further elongate under physiologic loads, (most pronounced when the inferior offset exceeds 2.5 mm) and significantly decreasing the yield displacement of the construct.
Keywords: Baseplate and glenosphere position, cadaver study, deltoid tension, failure, reverse total shoulder arthroplasty
How to cite this article: Wright J, Potts C, Smyth MP, Ferrara L, Sperling JW, Throckmorton TW. A quantitative analysis of the effect of baseplate and glenosphere position on deltoid lengthening in reverse total shoulder arthroplasty. Int J Shoulder Surg 2015;9:33-7 |
How to cite this URL: Wright J, Potts C, Smyth MP, Ferrara L, Sperling JW, Throckmorton TW. A quantitative analysis of the effect of baseplate and glenosphere position on deltoid lengthening in reverse total shoulder arthroplasty. Int J Shoulder Surg [serial online] 2015 [cited 2016 Sep 20];9:33-7. Available from: http://www.internationalshoulderjournal.org/text.asp?2015/9/2/33/154752 |
Introduction | |  |
Reverse total shoulder arthroplasty (RTSA) has revolutionized the treatment of rotator cuff-deficient shoulders, and its indications continue to expand to areas such as failed shoulder arthroplasty, revision arthroplasty, fracture sequelae, rheumatoid arthritis, instability and tumors. [1],[2],[3],[4] Due to these expanding clinical applications, the number of RTSA performed in the United States has increased dramatically since 2004. [5] Successful outcomes ideally maximize range of motion and minimize instability and complications. [6] Central to that success is the nonanatomic biomechanics of the prosthesis and the effectiveness of the deltoid to restore shoulder function with a deficient rotator cuff. [7] Studies have shown that the deltoid generates over 50% of the force necessary to elevate the arm in the scapular plane and is the only muscle remaining in cuff-deficient shoulders to power abduction in the same plane. [8] Ackland et al. found that increased abductor moment arms for anterior, middle, and posterior regions of deltoid assist to overcome cuff deficiency in RTSA. [9]
Reverse total shoulder arthroplasty constructs provided a stable and fixed fulcrum for elevation and increased resting length/tone of the deltoid. [7],[10] Optimizing deltoid tension is important to achieve maximal function, and lengthening of the deltoid increases the patient's ability to forward elevate, likely by recreating the force-length relationship of the deltoid muscle. [11] Intraoperative determination of deltoid tension is difficult and mostly guided by surgical experience. [7] Inferior glenosphere placement also has been found to decrease scapular notching and improve forward elevation by lengthening the deltoid. [12] This has prompted questions regarding the deltoid's ability to tolerate these increased stretch forces and may have implications for implant longevity. Until date, no studies quantifying the effect of baseplate and glenosphere position on deltoid tension exist.
The primary objective of this study was to quantify deltoid elongation under physiologic loads for three different baseplate-glenosphere configurations with increasing inferior offset. The secondary objective was to quantify elongation to failure (yield displacement) and to record the mode of failure. We hypothesized that the increased deltoid tension caused by increasing the inferior offset >2.5 mm would result in a lessened ability of the deltoid to further elongate under physiologic loads.
Materials and methods | |  |
Specimen preparation
Twenty-four fresh-frozen cadaver shoulders were divided into three groups of eight. The specimens were acquired from the Medical Education and Research Institute, Memphis, TN. Specimens were stored at −20°C. Before component implantation, the supraspinatus and infraspinatus of each specimen were sectioned to approximate a rotator cuff-deficient shoulder. The subscapularis, teres minor, pectoralis major, latissimus dorsi, and deltoid tendons were left intact. This is consistent with specimen preparation in other cadaver studies involving RTSA. [9],[11],[13],[14],[15]
The components were implanted according to the manufacturer's specifications (Biomet Comprehensive Shoulder System, Warsaw, IN). A starting point for the humeral stem was made in the rotator cuff footprint and enlarged with a burr. Starting with the opening reamer (4 mm), reaming proceeded sequentially in 1 mm increments until solid cortical contact was felt in the intramedullary canal. The humeral cutting guide was applied, and the humeral head was cut in 30° of retroversion. Broaching then proceeded sequentially from the 4 mm broach to the same size as the reamed diameter. The corresponding humeral stem was then impacted into position.
In group 1, the starting point for baseplate insertion was the center of the glenoid, which was identified based on the measured midpoint between the superior/inferior and the anterior/posterior margins of the glenoid. Glenospheres were placed in minimal inferior offset (0.5 mm). Group 2 baseplates were placed 2 mm inferior to the center point and glenospheres were placed in minimal offset (2.5 mm total inferior offset) [Figure 1]. Group 3 baseplates were placed in the same inferior position with glenospheres placed in maximal inferior offset (4.5 mm total inferior offset). Using a template with a 10° the inferior tilt built in, a guide pin was placed. The cannulated glenoid reamer was then used to plane the glenoid surface in 10° of the inferior tilt. The glenoid baseplate was inserted and impacted into position based on the previously described groups. Using a depth gauge, the length of the 6.5 mm center screw was measured, and the corresponding screw was placed. Four peripheral locking screws were then placed. All screws were placed in bicortical fashion for maximal fixation, with lengths varying by the size of the glenoid vault. A 36 mm glenosphere was impacted into position, again based on the parameters described for each group. A +0 mm polyethylene bearing surface was impacted into position in each specimen. After implantation, the glenohumeral joint was reduced, and all specimens were surveyed visually to inspect their condition.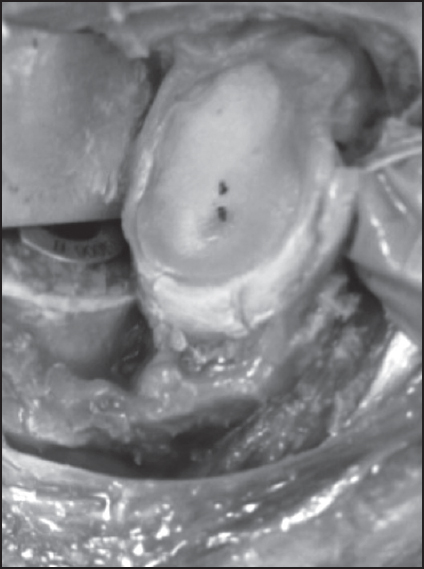 | Figure 1: The starting point for baseplate insertion in Group 1 was the center of the glenoid, which was identified based on the measured midpoint between the superior/inferior and anterior/posterior margins of the glenoid. Baseplates in Groups 2 and 3 were placed 2 mm inferior to the center of the glenoid
Click here to view |
Biomechanical testing
Tensile testing was done on all 24 specimens to quantify deltoid elongation and evaluate failure. To aid in gripping the specimen, the scapula was embedded in polyester resin. The scapula was oriented in the resin such that the shoulder was aligned in an anatomical "at rest" position when mounted to the actuator. Physiologic tension was placed on latissimus dorsi (15 N) and pectoralis major (15 N) tendons by way of pulleys and hanging weights [Figure 2]. This is consistent with specimen preparation in other studies of reverse shoulder arthroplasty. [9],[13],[14],[15],[16] Each specimen was placed into resting position, and the deltoid elongation was measured by pulling the humerus in tension to 30 N at a rate of 5 mm/min. The machine then maintained 30 N of tension for 5 min and recorded the elongation. Next, the specimen was pulled in tension at a rate of 10 mm/min until failure of the deltoid or RTSA components was observed.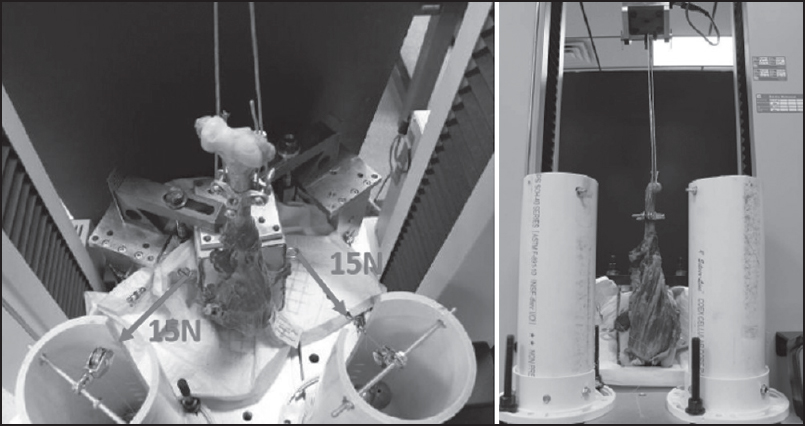 | Figure 2: Test apparatus with shoulder specimen embedded in resin with pulley system arranged to apply constant physiologic loads
Click here to view |
Data analysis
The tensile load and displacement data recorded for each specimen were analyzed in TestWorks 4 (MTS 2004). The test method was configured to calculate the yield load, yield displacement, peak load, and peak displacement.
Statistical analysis was conducted to provide a comparison among treatment groups with respect to the deltoid elongation, percent of elongation, and yield load observed. A one-way analysis of variance (ANOVA) was performed to detect statistically significant differences among treatment groups. A post-hoc Neuman-Keul's comparison was conducted to perform discrete comparisons among treatment groups after the performed ANOVA was found to be significant. Differences with P < 0.05 were considered as significant.
Results | |  |
For all specimens, the average deltoid elongation was 1.1 mm (0.4 mm to 2.6 mm), with an average yield load to failure of 582.5 N (range 305.9 N to 848.3 N) and an average peak load of 864.1 N (range 431.2 N to 1747.7 N). No significant difference in deltoid yield load was found among groups (P > 0.05) [Table 1].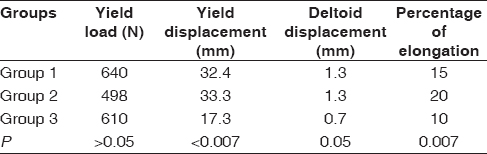 | Table 1: Significant changes in deltoid yield displacement and percentage of elongation after inferior offset exceeded 2.5 mm
Click here to view |
Deltoid elongation after loading decreased with increasing inferior offset of >2.5 mm. The average deltoid elongation after loading was 1.3 ± 0.7 mm for Group 1, 1.3 ± 0.5 mm for Group 2, and 0.7 ± 0.2 mm for Group 3 (P = 0.05). The percent of elongation of the deltoid also was decreased significantly between groups 2 and 3 (20% vs. 10%, P = 0.007). Deltoid displacement at failure (yield displacement) decreased from 33.3 mm for Group 2-17.3 mm for Group 3 (P = 0.007).
Sixteen of the 24 specimens (67%) failed by anterior deltoid detachment from the acromion [Figure 3]. Other modes of failure included deltoid tendon failure (16%), specimen pull-out of the polyester resin (8%), distal acromion fracture (4%), and humeral implant loosening (4%).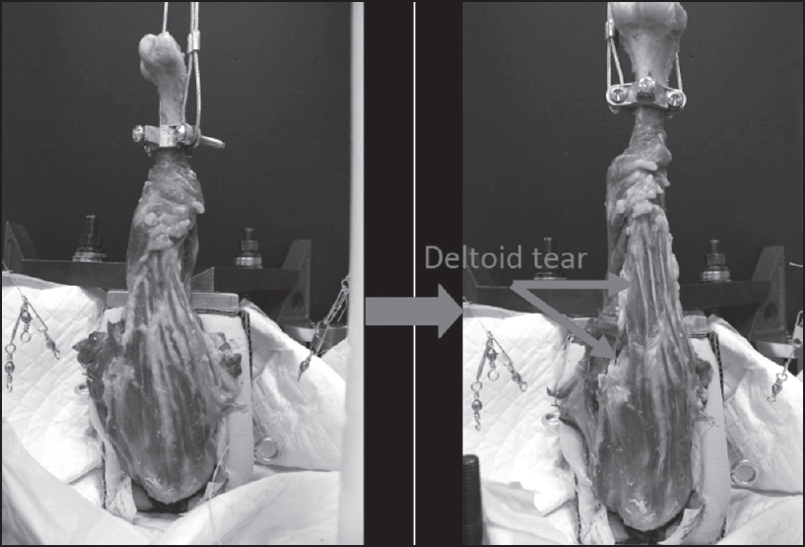 | Figure 3: Specimen after load to failure showing anterior deltoid detachment
Click here to view |
Discussion | |  |
Intuitively, deltoid tension is increased by increasing inferior baseplate and/or glenosphere offset. RTSA takes advantage of this tension by increasing the deltoid moment arm, and an inferior baseplate position has been shown to increase the efficiency of the deltoid up to 30%. [3],[4],[6],[9],[15],[17],[18],[19] Previous studies have shown inferior baseplate position and glenosphere tilt are protective against scapular notching with improved function, more uniform compressive forces with decreased shear force on the bone-baseplate interface and greater range of motion before impingement. [12],[20],[21],[22] The ability of the deltoid to accommodate lengthening has not been quantified.
Conceptually, the muscle tension-elongation relationship is set by Blick's curve, which suggests that lengthening of a muscle results in increased tension but at a certain tension, the muscle will no longer be able to lengthen. [23] Our study suggests that RTSA constructs with inferior offset of >2.5 mm diminish the ability of the deltoid to further elongate under physiologic loads. This was most pronounced between Groups 2 and 3 where total inferior offset of the constructs increased from 2.5 mm to 4.5 mm. When comparing these two groups, deltoid elongation under physiologic loading conditions decreased from 1.3 mm to 0.7 mm. While this represents merely a 0.6 mm difference in absolute terms, it reflects the diminished ability of the deltoid to tolerate increasing tension, effectively demonstrating Blick's curve. Further, increasing inferior offset significantly decreased the percent of elongation between these two groups. Most significantly, the deltoid yield displacement of 33.3 mm in Group 2 dropped to 17.3 mm in Group 3. This suggests a dramatic change in the ability of the deltoid to accommodate physiologic loads when inferior offset is increased from 2.5 mm to 4.5 mm. Essentially, at the tension generated with 4.5 mm of inferior offset, the deltoid can lengthen only an additional 17 mm before failing compared to an additional 33 mm when the RTSA construct is set at 2.5 mm of inferior offset.
Increased deltoid force may have clinical implications after RTSA, such as increased recovery length or increased risk of acromial stress fracture. [15],[24] Increased deltoid forces over a longer period may lead to deltoid-related pain and accelerate the decline in function that has been reported at mid-term follow-up. [15],[25] In our study, the most common mode of deltoid failure in these nonpathologic specimens was detachment of the anterior deltoid from the acromion, which may lead to increased pain and functional loss. [9] In a case series of anterolateral deltoid ruptures following RTSA in a group who had previous open RTC repairs, all patients had significant declines in functional outcome after rupture. [8] Anterior deltoid insufficiency also has been linked to instability and is underestimated in revision surgery. [26] Postoperative fractures of the acromion and scapular spine are rare, [26] and our study's 4% rate of acromion fracture is similar to that of previous studies. [27],[28] While this particular complication remains uncommon, function decreases significantly after fracture. [29]
As with other biomechanical and cadaver studies, recognized limitations exist. Mechanical actuators and static loads could not simulate active muscle contraction, proprioceptive control, and dynamically changing muscle lines of action. [6] The cadaver model with the scapula locked statically in resin was unable to account for the increased scapulothoracic motion and altered kinematics in RTSA. [30] Furthermore, the specimens used in this study were not pathologic and may not precisely mimic the degenerative changes seen in most patients who have RTSA. Finally, while we used baseplate and glenosphere positions as the primary variables to increase deltoid tension, these are not the only two factors involved in setting deltoid tension in RTSA. We speculate that any configuration that increases deltoid tension (including humeral-sided factors such as the size of the head cut or augmented humeral bearing trays) may well result in similar changes.
Increasing inferior offset in RTSA constructs appears to increase stretch forces on the deltoid. This results in a progressively diminished ability of the deltoid to further elongate under physiologic loads and is most pronounced when the inferior offset exceeds 2.5 mm. This configuration also significantly decreases the yield displacement of the construct, suggesting that the deltoid may not tolerate this additional lengthening to the same extent as with other less-tensioned constructs; however, this potential disadvantage may be balanced by the ability to minimize scapular notching with inferiorly offset configurations.
References | |  |
1. | Clark JC, Ritchie J, Song FS, Kissenberth MJ, Tolan SJ, Hart ND, et al. Complication rates, dislocation, pain, and postoperative range of motion after reverse shoulder arthroplasty in patients with and without repair of the subscapularis. J Shoulder Elbow Surg 2012;21:36-41. |
2. | Drake GN, O′Connor DP, Edwards TB. Indications for reverse total shoulder arthroplasty in rotator cuff disease. Clin Orthop Relat Res 2010;468:1526-33. |
3. | Mulieri P, Dunning P, Klein S, Pupello D, Frankle M. Reverse shoulder arthroplasty for the treatment of irreparable rotator cuff tear without glenohumeral arthritis. J Bone Joint Surg Am 2010;92:2544-56. |
4. | Nam D, Kepler CK, Neviaser AS, Jones KJ, Wright TM, Craig EV, et al. Reverse total shoulder arthroplasty: Current concepts, results, and component wear analysis. J Bone Joint Surg Am 2010;92 Suppl 2:23-35. |
5. | Kim SH, Wise BL, Zhang Y, Szabo RM. Increasing incidence of shoulder arthroplasty in the United States. J Bone Joint Surg Am 2011;93:2249-54. |
6. | Henninger HB, Barg A, Anderson AE, Bachus KN, Tashjian RZ, Burks RT. Effect of deltoid tension and humeral version in reverse total shoulder arthroplasty: A biomechanical study. J Shoulder Elbow Surg 2012;21:483-90. |
7. | Boileau P, Watkinson DJ, Hatzidakis AM, Balg F. Grammont reverse prosthesis: Design, rationale, and biomechanics. J Shoulder Elbow Surg 2005;14:147S-61. |
8. | Whatley AN, Fowler RL, Warner JJ, Higgins LD. Postoperative rupture of the anterolateral deltoid muscle following reverse total shoulder arthroplasty in patients who have undergone open rotator cuff repair. J Shoulder Elbow Surg 2011;20:114-22. |
9. | Ackland DC, Roshan-Zamir S, Richardson M, Pandy MG. Moment arms of the shoulder musculature after reverse total shoulder arthroplasty. J Bone Joint Surg Am 2010;92:1221-30. |
10. | Matsen FA 3 rd , Boileau P, Walch G, Gerber C, Bicknell RT. The reverse total shoulder arthroplasty. J Bone Joint Surg Am 2007;89:660-7. |
11. | Jobin CM, Brown GD, Bahu MJ, Gardner TR, Bigliani LU, Levine WN, et al. Reverse total shoulder arthroplasty for cuff tear arthropathy: The clinical effect of deltoid lengthening and center of rotation medialization. J Shoulder Elbow Surg 2012;21: 1269-77. |
12. | Simovitch RW, Zumstein MA, Lohri E, Helmy N, Gerber C. Predictors of scapular notching in patients managed with the Delta III reverse total shoulder replacement. J Bone Joint Surg Am 2007;89:588-600. |
13. | Ackland DC, Richardson M, Pandy MG. Axial rotation moment arms of the shoulder musculature after reverse total shoulder arthroplasty. J Bone Joint Surg Am 2012;94:1886-95. |
14. | Gulotta LV, Choi D, Marinello P, Knutson Z, Lipman J, Wright T, et al. Humeral component retroversion in reverse total shoulder arthroplasty: A biomechanical study. J Shoulder Elbow Surg 2012;21:1121-7. |
15. | Henninger HB, Barg A, Anderson AE, Bachus KN, Burks RT, Tashjian RZ. Effect of lateral offset center of rotation in reverse total shoulder arthroplasty: A biomechanical study. J Shoulder Elbow Surg 2012;21:1128-35. |
16. | Schwartz DG, Kang SH, Lynch TS, Edwards S, Nuber G, Zhang LQ, et al. The anterior deltoid′s importance in reverse shoulder arthroplasty: A cadaveric biomechanical study. J Shoulder Elbow Surg 2013;22:357-64. |
17. | De Wilde L, Audenaert E, Barbaix E, Audenaert A, Soudan K. Consequences of deltoid muscle elongation on deltoid muscle performance: A computerised study. Clin Biomech (Bristol, Avon) 2002;17:499-505. |
18. | Grammont PM, Baulot E. Delta shoulder prosthesis for rotator cuff rupture. Orthopedics 1993;16:65-8. |
19. | Terrier A, Reist A, Merlini F, Farron A. Simulated joint and muscle forces in reversed and anatomic shoulder prostheses. J Bone Joint Surg Br 2008;90:751-6. |
20. | Gutiérrez S, Comiskey CA 4 th , Luo ZP, Pupello DR, Frankle MA. Range of impingement-free abduction and adduction deficit after reverse shoulder arthroplasty. Hierarchy of surgical and implant-design-related factors. J Bone Joint Surg Am 2008;90:2606-15. |
21. | Gutiérrez S, Greiwe RM, Frankle MA, Siegal S, Lee WE 3 rd . Biomechanical comparison of component position and hardware failure in the reverse shoulder prosthesis. J Shoulder Elbow Surg 2007;16:S9-S12. |
22. | Nyffeler RW, Werner CM, Gerber C. Biomechanical relevance of glenoid component positioning in the reverse Delta III total shoulder prosthesis. J Shoulder Elbow Surg 2005;14:524-8. |
23. | Salter, RB. Textbook of Disorders and Injuries to the Musculoskeletal System. Baltimore, MD: Lippincott Williams and Wilkins; 1999. p. 25-6. |
24. | Levy JC, Anderson C, Samson A. Classification of acromial stress fractures following reverse total shoulder arthroplasty. J Bone Joint Surg Am 2013;95:e1041-7. |
25. | Guery J, Favard L, Sirveaux F, Oudet D, Mole D, Walch G. Reverse total shoulder arthroplasty. Survivorship analysis of eighty replacements followed for five to ten years. J Bone Joint Surg Am 2006;88:1742-7. |
26. | Zumstein MA, Pinedo M, Old J, Boileau P. Problems, complications, reoperations, and revisions in reverse total shoulder arthroplasty: A systematic review. J Shoulder Elbow Surg 2011;20:146-57. |
27. | Lädermann A, Williams MD, Melis B, Hoffmeyer P, Walch G. Objective evaluation of lengthening in reverse shoulder arthroplasty. J Shoulder Elbow Surg 2009;18: 588-95. |
28. | Werner CM, Steinmann PA, Gilbart M, Gerber C. Treatment of painful pseudoparesis due to irreparable rotator cuff dysfunction with the Delta III reverse-ball-and-socket total shoulder prosthesis. J Bone Joint Surg Am 2005;87:1476-86. |
29. | Wahlquist TC, Hunt AF, Braman JP. Acromial base fractures after reverse total shoulder arthroplasty: Report of five cases. J Shoulder Elbow Surg 2011;20:1178-83. |
30. | Kwon YW, Pinto VJ, Yoon J, Frankle MA, Dunning PE, Sheikhzadeh A. Kinematic analysis of dynamic shoulder motion in patients with reverse total shoulder arthroplasty. J Shoulder Elbow Surg 2012;21:1184-90. |
[Figure 1], [Figure 2], [Figure 3]
[Table 1]
|